
Abstract
Can dirt help fight superbugs? Students and staff at UCOL dug into their backyards to find out. As part of the Tiny Earth antibiotic discovery project, they collected 12 soil samples and isolated 137 bacteria. Testing revealed 40 bacteria that could fight multiple harmful pathogens, including Escherichia coli and Staphylococcus aureus. Chemical extractions were performed on the 10 best performing strains, uncovering potential antibiotics. Future work will optimise antibiotic-producing conditions and current students will fine tune methods to purify and chemically characterise these natural products.
Introduction
Antibiotics are structurally diverse molecules that often feature complex scaffolds including heterocycles, chiral centres, and a variety of functional groups such as hydroxyls, ketones, or amides.1 Their complex structures allow them to interact with specific bacterial targets, such as enzymes or ribosomal subunits, allowing them to disrupt essential cellular processes to either prevent the bacteria from replicating or cause their death.2 In penicillin, the β-lactam ring inhibits bacterial cell wall synthesis, while Erythromycin has a macrocyclic lactone ring that interferes with protein synthesis.2 Penicillin and Erythromycin belong to different classes of antibiotics of which there are 18 major classes.3 The structural complexity and variation of antibiotics not only underpins their biological activity but also shows the potential for microorganisms to produce useful and diverse natural products.4
The rise of antibiotic resistance presents a critical threat to global health, and the future of modern medicine depends on effective antibiotics. Pathogens such as Escherichia coli, Klebseilla pneumoniae, and Staphylococcus aureus lead the mortality burden.5 As bacteria continue to evolve resistance mechanisms against available antibiotics, the development of novel compounds with new mechanisms of action is essential to maintain effective treatment strategies.6 Historically, soil has been a fertile ground for antibiotic discovery.7 Antibiotics are the products of secondary metabolism; where microorganisms repurpose their primary metabolites to generate complex organic compounds.8 Bacteria which have the capability to produce antibiotics will not produce them all the time and may only produce them under certain conditions.9
Culturing soil microbes for antibiotic discovery has unique challenges as many organisms require a complex and often nutrient-poor environment to grow.10 Traditional nutrient rich media like Plate Count Agar (PCA) are excellent for cultivating many fast-growing bacteria but it can often inhibit or outcompete the growth of the diverse and often slow-growing microbes found in soil.11 It is often the slower growing organisms which are the source of novel secondary metabolites, including antibiotics.12 Reasoner's 2A (R2A) agar is a low-nutrient medium specifically formulated to mimic the low nutrient conditions of soil and water which allows for the growth of a broader range of soil microbes, including slow-growing and fastidious species that may be missed on PCA.11 In this study, both PCA and R2A agar are used to increase the likelihood of capturing both slow and fast growing microbes and in turn uncovering novel antibiotic producers. The two methods are statistically compared to guide media choice in further studies.
In order to identify and understand the natural products produced by soil isolates, chemical extraction and characterisation are needed.13 Extraction methods are used to isolate natural products from either agar or broth that the bacteria grow in.14 Solvent-based extraction methods separate bioactive compounds from microbial cultures, while chromatographic techniques such as thin-layer chromatography (TLC) and high-performance liquid chromatography (HPLC) enable separation and purification of individual components.15 Advanced spectroscopic methods like nuclear magnetic resonance (NMR) and mass spectrometry (MS) then provide detailed structural insights, facilitating the identification of known antibiotics or discovery of novel compounds.12
New Zealand is geographically isolated with diverse landscapes and habitats and therefore holds promise for discovery of microbes with specialised adaptations and potentially novel antibiotic production capabilities.16 Tiny Earth (TE; https://tinyearth.wisc.edu/) was established to address the antibiotic crisis through a student crowdsourcing research initiative and there are participating institutes worldwide. This article describes the isolation of microorganisms from soil, screening them for antibiotic activity, and identifying the most promising isolates capable of producing bioactive compounds. The study further focuses on extracting the natural products produced by these isolates, laying the groundwork for future research into optimising growth conditions, refining extraction methods, and characterising products to contribute to the antibiotic discovery pipeline.
Materials and methods
Soil sample collection
Twelve soil samples were collected from diverse locations around Palmerston North and Foxton Beach in New Zealand, including gardens, creek banks, and areas beneath trees. GPS coordinates and soil temperatures are listed in Table 1. Samples of approximately 10-20 g were taken at a depth of 5–10 cm using sterile plastic tubes, labelled numerically (1–12), and stored at 4°C prior to analysis. Not all participants measured soil temperatures. All participants signed an informed consent document and took only what was needed and collected samples from their own private property. Appropriate tikanga principles in soil collection were discussed with participants.
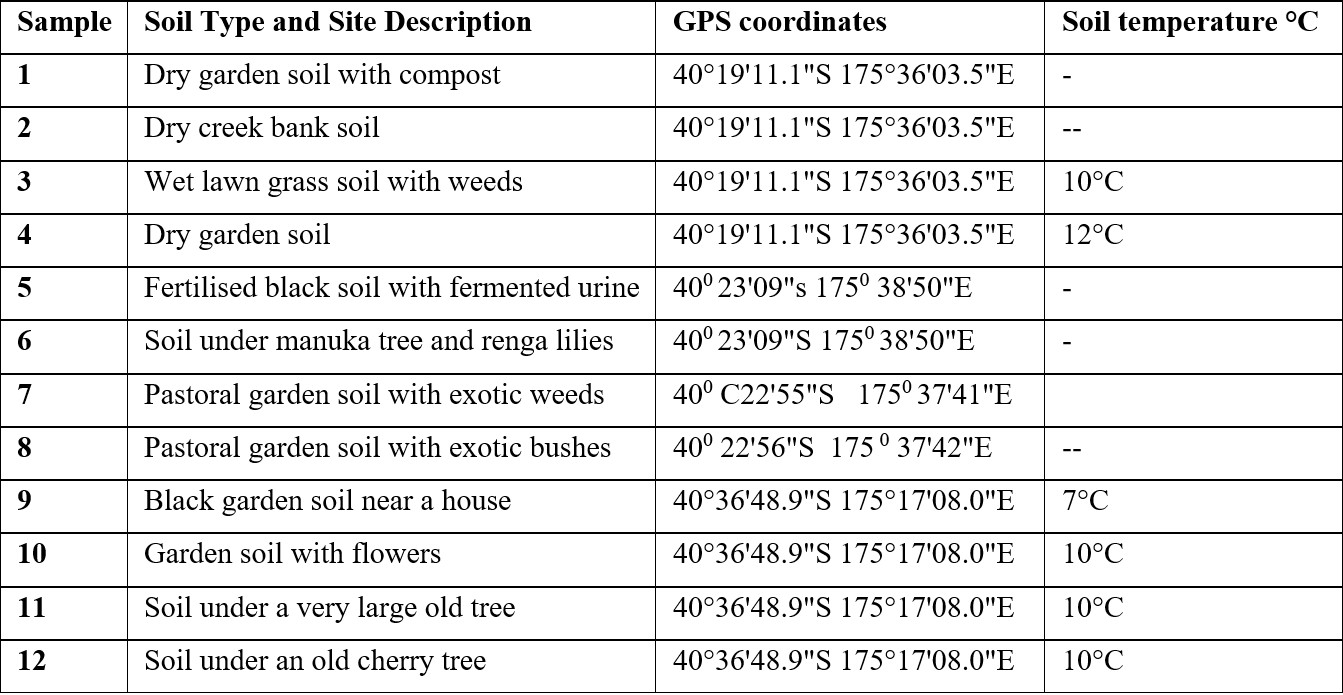
Isolation of culturable microbes
Soil bacteria were isolated using the serial dilution and plating technique. Briefly, 1 g of each soil sample was weighed and suspended in 10 mL of deionised water by vortexing for 5 minutes at maximum speed. The samples were then serially diluted and 100 µL from each dilution of each sample was used to inoculate agar plates. R2A and PCA were used as growth media. The plates were incubated for 7 days at 25°C, colonies were counted and unique colonies in each sample were sub-cultured for further testing and analysis. The number of colony forming units (CFU) per gram of soil was calculated for each sample based on plate dilutions. The number of isolates per sample was also recorded.
Primary screening
Petri plates were preinoculated with the target organisms using a cotton swab. Potential antibiotic producing isolates were then transferred onto PCA agar plates to create small patches (6–10 mm). On each plate, 16–20 patches of different isolates were tested. Plates were incubated at 37°C for 7 days. Test organisms used were: Enterococcus faecalis (ATCC14505), Staphylococcus aureus (ATCC25923), Escherichia coli (ATCC25922), Acinetobacter baylyi (ATCC 33305), Pseudomonas aeruginosa (ATCC27853) and Serratia marcescens (ATCC13880).
Extraction of secondary metabolites
Ten promising isolates were swabbed onto four R2A plates each. Plates were incubated at 25°C for 7 days until dense growth was observed. The agar plates were cut into small 2 cm square pieces and placed in a bottle. The bottles were frozen at -20°C overnight, thawed, and extracted with 10 mL ethyl acetate and 5 mL distilled water. The organic layer was separated by centrifugation (2000 rpm, 10 minutes), dried with sodium sulfate, and concentrated using rotary evaporation.
Biochemical and morphological analysis
Biochemical and morphological tests were performed on the 10 promising isolates. Catalase, oxidase, coagulase, sheeps blood agar hemolysis, and motility tests were done according to standard protocols.17 Gram stains were also done and stain reaction as well as morphology were recorded.
Antimicrobial assay
Ethyl acetate extracts were evaluated for antibacterial activity using the Kirby-Bauer disc diffusion method. Plates containing 20 mL of agar were inoculated with pathogens as described in primary screening, and sterile discs loaded with 10 µL of each extract were placed on the agar surface. Positive (Ampicillin, Clindamycin, Ciprofloxacin, Trimethoprim) and negative (ethyl acetate) controls were included. After incubation at 37°C overnight, inhibition zones were measured in millimetres. All tests were performed in triplicate.
Statistical analysis
To compare the effectiveness of different media types (PCA and R2A), a two-tailed student’s t-test in Microsoft Excel (Microsoft Excel, 2016) was used to compare both CFU per gram and colony types per sample.
Results
R2A agar supported significantly higher colony growth (p < 0.01) per gram of soil than PCA, as shown in Fig. 1a. R2A agar was also able to grow a far greater (p < 0.01) variety of colony morphology types as shown in Fig. 1b. Across the 12 samples, 137 isolates were identified and patched onto new plates for further testing. 108 came from R2A and 29 came from PCA plates.
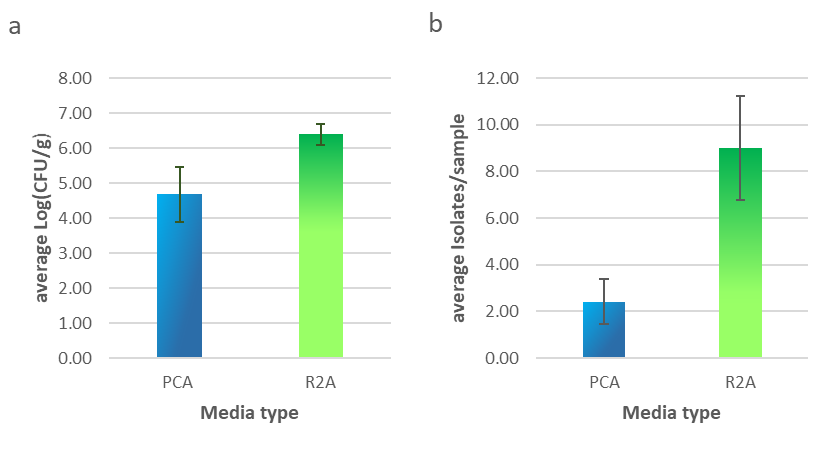
To identify antagonist activity, a spread patch method was used against the following pathogens: Enterococcus faecalis, Staphylococcus aureus, Escherichia coli, Acinetobacter baylyi, Pseudomonas aeruginosa and Serratia marcescens. Primary screening showed 102 isolates with some antibiotic activity against at least one of the pathogens tested. 93 of these were from R2A plates, and 9 were from PCA plates. Isolates were classed as broad spectrum if they showed activity against both gram negative and gram positive pathogens. 40 isolates showed this activity and all were from R2A plates. The samples from which isolates came are detailed in Table 2. Out of the 40 isolates that showed broad spectrum activity, 10 were chosen based on the size of the zone of inhibition and the number of pathogens they were active against for antibiotic extraction. The soil samples these isolates came from were sample numbers 1, 3, 4, 5, 6, 7, and 11. Two isolates each were isolated from samples 3, 4 and 11. After evaporation, 3 of the 10 isolates yielded products.

The 10 chosen isolates were characterised based on their morphology, metabolism and motility. All of them were gram positive rod shaped, spore forming, catalase positive and coagulase negative. Details of these tests are shown in Table 3. The naming system for the isolates follows the format S (sample number) – (isolate number from that sample). Some of the motility agar results were difficult to interpret and have been listed as ‘unclear’ indicating that the test was not able to identify whether the bacteria were motile or not.
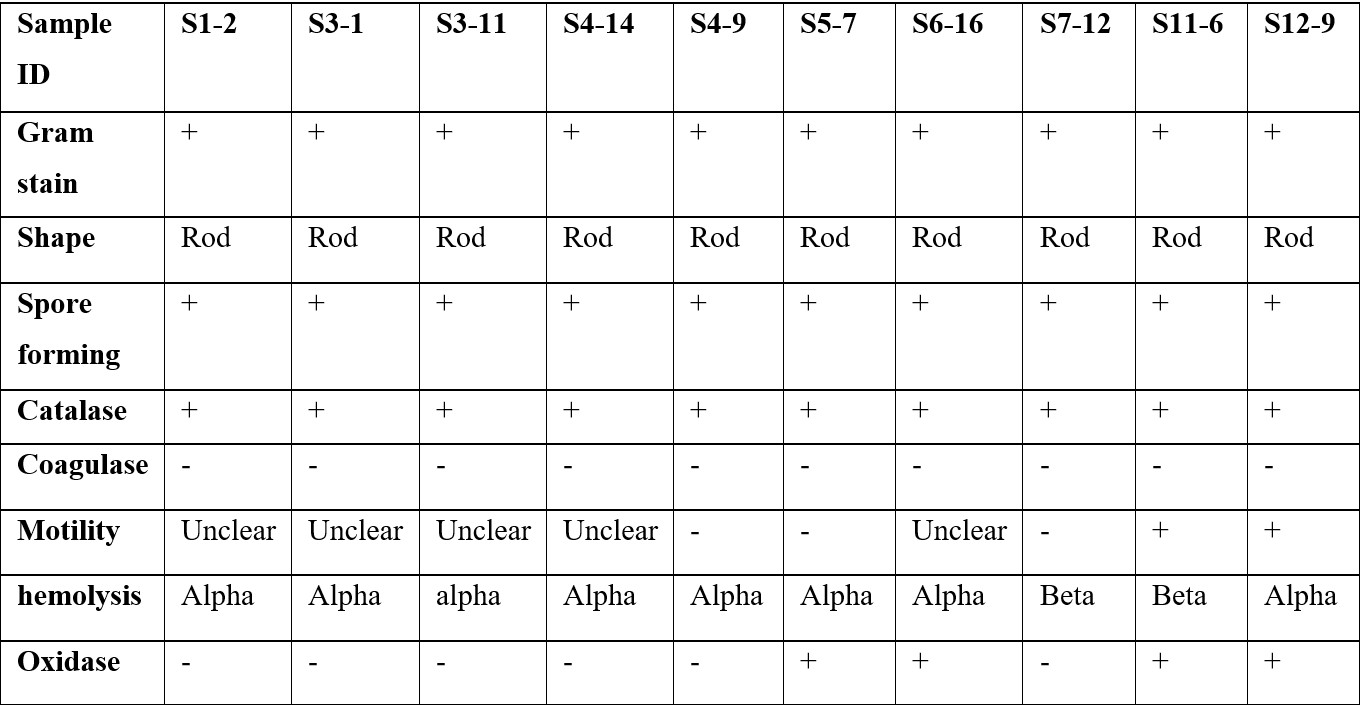
Discussion
This study demonstrates that soil microorganisms remain a promising source of antibiotics. The isolated strains exhibit significant antimicrobial activity and provide a basis for future investigations to characterise both the strains and metabolites. The comparison of different media types showed that most of the antibiotic producers came from the low nutrient R2A agar and all of the most promising strains came from organisms isolated from this type of agar. The difference between both CFU/g and number of colony types per sample was statically significant (p < 0.01) for both comparisons.
As sample sites were selected based on accessibility rather than ecological characteristics, this study does not provide direct insights into which environments are most likely to yield antibiotic-producing microorganisms. Samples 3 and 4 were from the same property and together they yielded 17 isolates with broad spectrum activity out of the total of 40 isolates. Sample 3 was from the lawn and sample 4 was from the garden on that property. Future experiments could compare different soil environments such as under different native trees or compare urban soil to native bush.
The CFU per gram of soil values were in a range similar to other studies, with Gislin et al.18 reporting a range from 280,000 to 6,300,000 in soil samples taken from around various crops using nutrient agar which is similar to PCA. Across their 10 soil samples they identified 8 organisms which showed antibiotic activity, which is similar to the 9 antibiotic producers in this study which were isolated from PCA.
Characterisation of the isolates showed results consistent with Abdukadir et al.19 who isolated gram positive bacilli. Further biochemical tests or 16S gene sequencing experiments are needed to definitively identify the isolates. It is possible based on the results shown that they belong to the phylum of Actinobacteria which are known for their clinically useful secondary metabolites.20
The extraction of metabolites from agar on 10 of our isolates yielded only 3 isolation products. Bacteria may only produce secondary products under certain conditions. The conditions for this experiment were incubation at 25°C for 1 week. Mohamed et al.12 used a coculture of pathogens and soil isolates to induce antibiotic production and Zhu et al.9 trialled 39 different conditions including changing nutrient composition, pH and salinity to elicit antibiotic production. Their results showed changes in pH to be the most efficient variable to modulate. Further trials of different growth conditions are planned for our 10 most promising isolates with the students who are doing research projects this year.
Chemical characterisation techniques such as HPLC and NMR spectroscopy will be key analytical tools in identifying the chemical composition of the secondary metabolites produced. HPLC will be used to purify and analyse the organic extracts based on a similar protocol reported by Singh et al.21 The resulting chromatograms will be compared against a library of known antibiotic standards to help identify the unknown compounds. It is expected that some chromatographic peaks will not match existing standards. Such samples will be subjected to further investigation to determine their structural and functional characteristics. Compounds showing strong antibacterial activity and obtained in sufficient quantities will undergo detailed structural analysis using NMR and mass spectrometry.22 These methods will enable further insight into the molecular structure and potential bioactivity of these new metabolites.
To supplement HPLC and NMR, UV-visible (UV-Vis) and infrared (IR) spectroscopy will also be employed. UV-Vis spectroscopy will help identify the chromophoric properties of the compounds, while IR spectroscopy will reveal information about their functional groups, contributing to a more complete molecular characterisation.22 In addition, an alternative extraction method using methanol as a solvent will be explored. This approach will complement the current ethyl acetate extraction method. Methanol, as a polar solvent, is expected to enhance the extraction of a broader spectrum of secondary metabolites, including those with different polarities.17 The methanol extracts will undergo the same analytical procedures to ensure consistent and reliable results and allow comparisons.
The findings in this study highlight the importance of low-nutrient media in isolating promising strains and emphasise the need for optimised growth conditions to enhance metabolite production. Conducted as part of an undergraduate research initiative, this work provided students with hands-on experience in microbiology and the continuation of the project will give the next round of students experience with analytical chemistry techniques while contributing to the broader effort to discover novel antibiotics. Future studies will refine extraction techniques, expand chemical analyses, and further characterise bioactive compounds, building on the initial screening for antibiotic producing isolates.
Acknowledgements
We thank Soumia Puthen and Morgan Ronchi Broadbent who undertook the bulk of the data collection as part of their laboratory project at the Universal College of Learning.
References
- Lewis, K. et al. Nature 2024, 632, 39–49.
- Hutchings, M. I.; Truman, A. W.; Wilkinson, B. Curr. Opin. Microbiol. 2019, 51, 72–80.
- Coates, A. R.; Halls, G.; Hu, Y. Br. J. Pharmacol. 2011, 163, 184–194.
- Quinn, G. A.; Dyson, P. J. npj Antimicrob. Resist. 2024, 2.
- Areda, D. et al. Lancet 2024, 404, 1199–1226.
- Lewis, K. Cell 2020 181, 29–45.
- Libz, F. Smithsonian 2020, 50(9), 42–53.
- Quinn, G. A.; Dyson, P. J. npj Antimicrob. Resist. 2024, 2.
- Zhu, H. et al. Microbiology (U.K.) 2014, 160, 1714–1726.
- Gauthier, L. K.; Foster, A.; Wagner, B. D.; Kirby, C. W. J. Vis. Exp. 2025.
- Reasoner, D. J.; Geldreich, E. E. Appl. Environ. Microbiol. 1985, 49.
- Mohamed, O. G. et al. Mar. Drugs 2021, 19.
- Moharkar, S.; Dhamole, P. B. Korean J. Chem. Eng. 2021, 38, 90–97.
- Iqbalsyah, T. M.; Nisak, C.; Sartika, F.; Saidi, N.; Febriani, F. IOP Conf. Ser.: Earth Environ. Sci. 2019, 276.
- Balagurunathan, R.; Selvameenal, L.; Radhakrishnan, M. Indian J. Pharm. Sci. 2009, 71, 499.
- Stott, M. B.; Taylor, M. W. N. Z. J. Ecol. 2016, 40.
- Cappuccino, J.; Sherman, N. Microbiology: A Laboratory Manual; Pearson, 2016.
- Gislin, D.; Sudarsanam, D.; Antony Raj, G.; Baskar, K. J. Genet. Eng. Biotechnol. 2018, 16, 287–294.
- Abdulkadir, M.; Waliyu, S. Eur. J. Appl. Sci. 2012, 4, 211–215.
- Rammali, S. et al. Sci. Rep. 2024, 14.
- Singh, V. et al. PLoS One 2018, 13, e0200500.
- Elyashberg, M. TrAC, Trends Anal. Chem. 2015, 69, 88–97.