Abstract
Ceaseless motion and change is central to the Chinese Tao philosophy, being evident in the representation of seasonal change trigrams, and the T’ai-chi T’u or Diagram of the Supreme Ultimate, where the continuous interplay between yin and yang could, in principle, result in an alternation of extremes, but actually results in a succession of compromises. Even in chemistry, the recognition that the different concentrations of the same element can be beneficial or hazardous is a manifestation of such compromise. The current efforts in ‘green chemistry’ and 'green engineering’ are attempts to reach compromises in chemical processing and energy sources and their efficiency that balance societal needs against excessive exploitation of natural resources and environmental degradation. Even though generally unrecognised as such, these initiatives are shown to be consistent with Taoist thought.
Introduction
An important feature of the Chinese Tao is ceaseless motion and change. However, such motion and change does not occur randomly, but cyclically. As Lao Tzu expressed it, “Returning is the motion of the Tao …. Going far means returning.” 1 Central to this cycle is the idea of polar opposites yin and yang, which set limits to the cycle of change.2 As the physicist Fritj of Capra expressed it:
“in the Chinese view, all manifestations of the Tao are generated by the dynamic interplay of these two polar forces. This idea is very old and many generations worked on the symbolism of the archetypal pair yin and yang until it became the fundamental concept of Chinese thought. The original meaning of the words yin and yang was that of the shady and sunny sides of a mountain, a meaning which gives a good idea of the relativity of the two concepts.” 3
In a further explanation, Capra goes on to say:
“From the very early times, the two archetypal poles of nature were represented not only by bright and dark, but also by male and female, firm and yielding, above and below. Yang, the strong male, creative power, was associated with Heaven, whereas yin dark receptive, female and maternal element, was represented by the Earth. Heaven is above and full of movement, the Earth – in the old geocentric view –is below and resting, and thus yang came to symbolize movement and yin rest. In the realm of thought, yin is the complex, female, intuitive mind, yang the clear and rational male intellect…”
Graham (1989) provided a list of yang terms and phrases.4 His ‘list A’ includes as examples: ’Heaven’, 'above’, ‘stretching’, ‘getting on in the world’ and their corresponding yin terms and phases. Graham’s ‘list B’ includes as examples: ‘Earth’, ‘below’, 'contracting’, and ‘being stuck where one is’, (corresponding respectively to those in ‘list A’), noting that: “…A is superior to B but the two are mutually dependent… As has long been recognised, China tends to treat opposites as complementary, [whereas] the West [treats them] as conflicting…”... and subsequently observing that: “There is indeed a rough similarity between the contrasts between A and B all down the table, enough to give meaning to ‘Yang’ and ‘Yin’, commonly described as the active and passive or the positive and negative principles… Therefore the Yang does to, [while] the Yin is transformed by.”5
The ancient Chinese symbol T’ai-chi T’u or Diagram of the Supreme Ultimate (Fig. 1) is a symmetric arrangement of the dark yin and the white yang, each of which is centred on a pole which rotates about the other, as shown in the sequence of photographs of an easily constructed model in Fig. 2. The important point to note in this sequence is that the shadows do not diminish as the diagram rotates; rather, they move past each other: ‘The yang returns cyclically to its beginning, the yin attains its maximum and gives place to the yang.’6 The consequence of this in Taoist terms is that there is an interplay of extremes in seasons, in health – in all of nature.
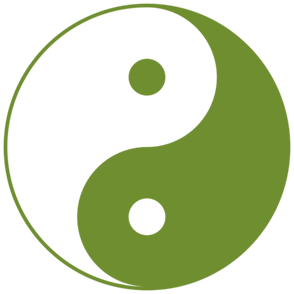
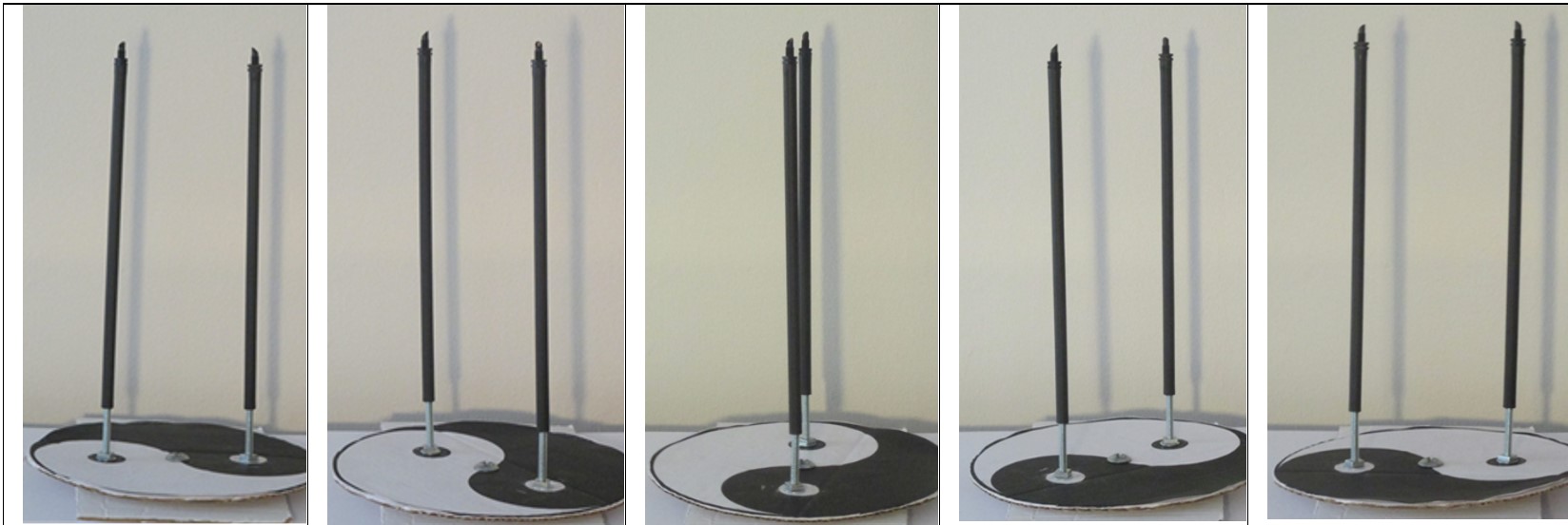
The interplay of yin and yang was portrayed in the I Ching by combinations of yin (represented as a broken line) and yang (represented by a solid line), called ‘trigrams’, each of which was associated with particular characteristics, including the cardinal points, the seasons, weather and family members (Fig. 3).7
Compromise in chemistry
Fig. 4 shows a modern interpretation of such an interplay: the compromise between toxicity and essentiality of the chemical elements to human health (hair in this particular example),8 shown on a version of the periodic table where the placement of elements is organised on the basis of their ionic potential (z/r, being the ratio of the ionic charge, z, to the ionic radius, r),9 justified because the biochemical reactions involved in the human body generally occur in aqueous solution and involve ionic species. In a similar way, some ions of the elements associated with gold processing are more toxic than other ions of the same element, as shown in Fig. 5,10 caused by the complexity of chemical reactions and ‘speciation’ (i.e., different ions and other entities) that are involved. Both examples suggest that ions of a given element may be both yin and yang.11
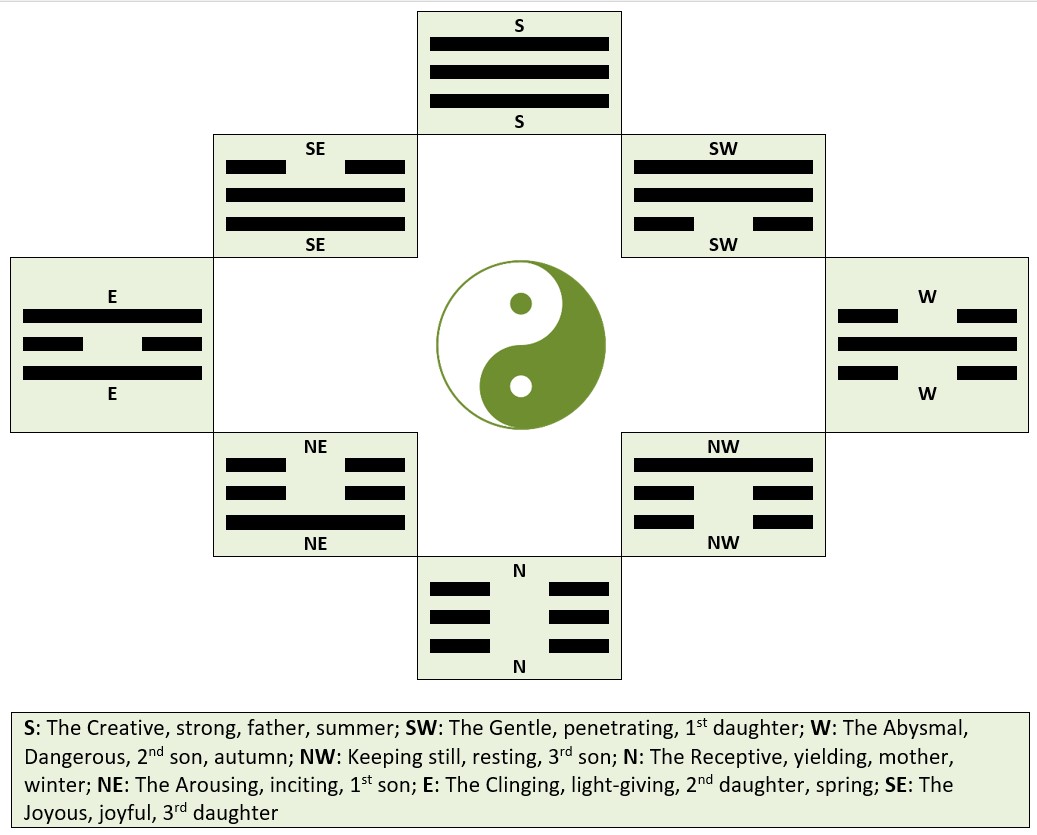

Fig. 4. Toxic and essential chemical elements in human hair,6 shown on the skeletal framework of the‘cation’ side of the ‘Earth scientist’s periodic table of the elements and their ions’ (based on Railsback 2018, p. 209, Fig. 11.19).

Fig. 5. Differentions of the same element associated with gold processing have different toxicities,10 as shown here on the skeletal framework of the ‘anion’ side of the ‘Earth scientist’s periodic table of the elements and their ions’ (based on Railsback 2018, p. 210, Fig. 11.29)
The rise of green chemistry
In recent years, 'green chemistry’ (or, as it is sometimes known, ‘sustainable chemistry’) has sought to reduce pollution and other adverse effects of chemical processes by increasing the efficiency of such processes through better design, manufacture and use of the chemicals involved. Whereas “green” movements generally advocate a complete change (e.g., the cessation of the use of fossil fuels for energy production and transportation, or the banning of plastic packaging), ‘green chemistry’ is more about attaining a compromise between exploitation and conservation or between utility and waste, or ‘good’ and ‘bad’ if you want to be colloquial, or – if you don’t think it too much of a stretch – between yang and yin, as suggested in Table 1.
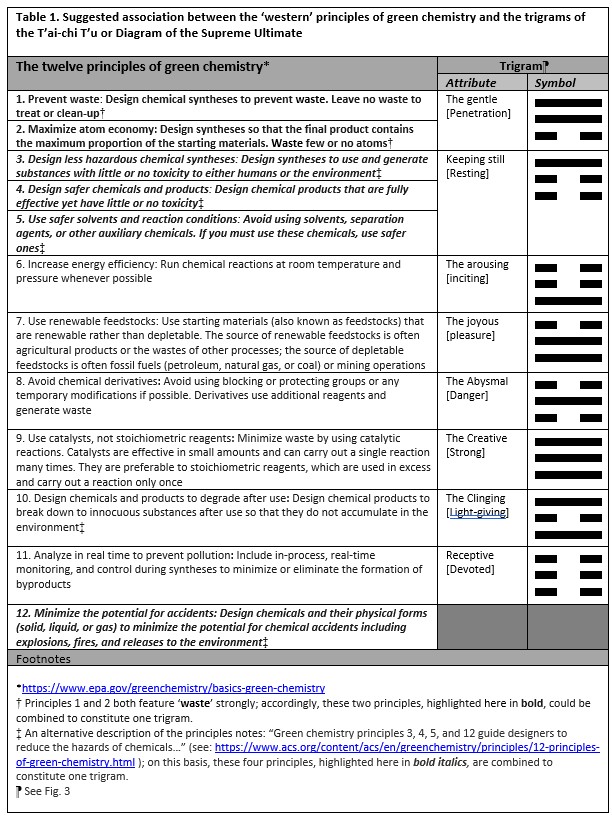
Environment: a wider context for a 'periodic table’ representation
An alternative to the ‘twelve principles’ alone that are shown in Table 1 is a scheme which has a passing similarity to the format of the Periodic Table of the chemical elements (Fig. 6).12
The analogies to the chemical elements are the techniques and processes that invoke ten of the twelve principles of green chemistry of Table 1. For each of these ‘elements’, Fig. 7 shows the total number of citations of the relevant references in Anastas and Zimmerman12 are also given. Citations of papers published are a measure of the impact of research: a high number of citations of a paper means that more researchers have referred to it in papers that they have published subsequently, and implies that its impact on the research community is more significant.

Fig. 6. The ‘elements’ of green chemistry and green engineering (modified from part of Anastas and Zimmerman, 2019, Fig. 112).
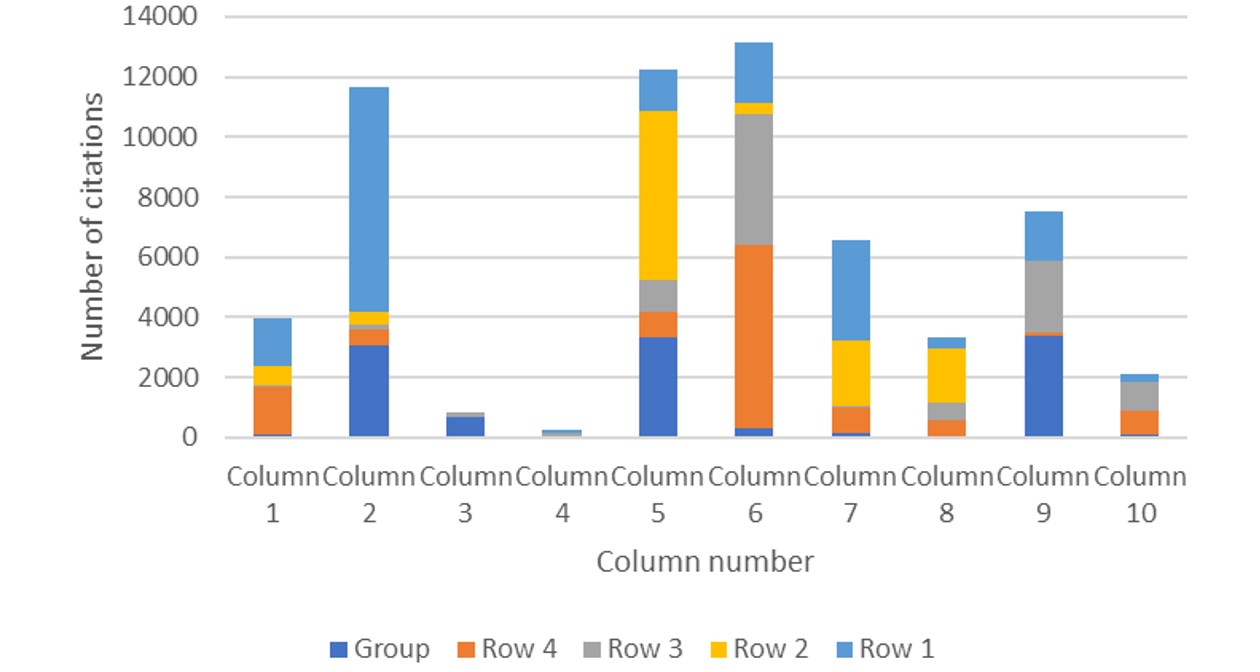
Fig. 7 shows that the engagement of scientists with the principles of green chemistry has been highly variable: the application of green chemistry to energy is the 'element’ (column 6) has the most citations, while application to molecular design (column 4) has the fewest citations. Catalysis (column 8) is much lower down the ‘pecking order’ of Fig. 7 than might be expected from Astruc’s13 observation that:
“… the heterogeneous metathesis reaction has been exploited by Schrock and Grubbs in an original way, involving the discovery and engineering of metal-alkylidenes and -alkylidynes to develop one of the most productive and useful catalytic reactions in chemistry. Metathesis is indeed presently used every day by the entire organic community, because of its extraordinary potential for the synthesis of molecular targets used as therapeutic agents and by polymer chemists for the synthesis of new materials, including biodegradable ones. By shortening synthetic paths and providing more facile access to therapeutic agents under increasingly more environmentally friendly catalytic conditions, metathesis now is at the forefront of “green chemistry”13
…especially given that the ‘metathesis’ method earned its discoverers – Yves Chauvin (of the Institut Français du Pétrole), Robert H. Grubbs (of CalTech), and Richard R. Schrock (of Massachusetts Institute of Technology) – the Nobel Prize in Chemistry in 2005.14
Only part of the scheme developed by Anastas and Zimmerman12 is shown in Fig. 6. The scheme also includes other ‘elements’ in columns that are labelled as ‘enabling strategies’ by which the principles of green chemistry and green engineering might be achieved as well as humanitarian and ‘noble’ goals, the latter of which were emphasised in a subsequent paper.15 Even so, green chemistry has been said to have “ignored social sustainability in its discourses, practices, and evaluations, leading to a reductionist interpretation of sustainability”, and which could be addressed by a more systems-based approach.16 Another commentary adds economics to the mix:
“… we must have positive perspectives for the future of green chemistry because it encompasses the future of our world. Green chemistry is not restricted to a chemical analysis in which a less toxic solvent is used. This is not green chemistry. Green chemistry is a set of actions and attitudes, it is multidimensional… It is thinking about the whole process and minimizes reagents, steps, costs and energy.
“…. industries need to visualize the economic viability of applying green chemistry to their processes, which prevents us from leveraging the use of this ideology. Investments and dissemination on the importance of green chemistry and how they affect directly from the start of pharmaceutical analyses, employees and patient health to environmental sustainability are extremely important for the process of future improvements.”17
Another recent approach towards developing a ‘green periodic table ’has been to combine four measures of sustainability, viz., ‘the Human Development Index (sourced from the Human Development Report), the Global Warming Potential (sourced from the CML 2001 environmental impact category methodology), the Recycling Rate and the National Economic Importance (both sourced from the United States Geological Survey)’, which were then “normalized, equally weighted and aggregated using a geometric aggregation methodology to provide a single quantitative measure of sustainability”.18 The results of this analysis are here presented in a traffic light visualisation (Fig. 8); the work also revealed that the element with the highest sustainability index is titanium. Although titanium’s recently developed use in drinking straws and incorporation into plastics bottles has environmental benefits,19 its sustainability index, nevertheless, has declined over the decade from 2005 to 2015, as have the other metals shown in Fig. 8.
Periodic tables such as that shown in Fig. 8 bring together the type of data that had previously been used to generate similar looking periodic tables that feature conservation of resources (e.g., Fig. 9)20 or environmental concerns. Comparison of Fig. 8 with Fig. 9 indicates that for many elements a compromise is indicated between sustainability and long-term availability. Fig. 10 shows an example of an integrated form of these two diagrams that highlights the combination for each element of the energy demands, the potential for global warming, terrestrial acidification, and freshwater eutrophication.21

Fig. 8. The cation side of the earth scientist’s periodic table of the elements and their ions (Railsback 2018, p.209, Fig. 11.19), showing the Chemical Element Sustainability Index (CESI) in a red-orange/amber-green ‘traffic light’ format for 2015. In this diagram, the rare earth elements and silicon have a low CESI, caused by the lack of recycling associated with these elements (Smith et al. 202118).

Fig. 9. The cation side of the earth scientist’s periodic table of the elements and their ions (Railsback 2018, p. 209, Fig. 11.19), showing estimates of the number of years before known reserves of elements are depleted (modified from Supanchaiyamat and Hunt 201820).

Fig. 10. The cation side of the earth scientist’s periodic table of the elements and their ions (Railsback 2018, p. 209, Fig. 11.19), showing the rating of a combination of environmental concerns related to elements: the energy demands, the potential for global warming, terrestrial acidification and freshwater eutrophication (compiled from Nuss and Eckelman,2014, Fig. 2 and Fig. 321)
Collectively, these three diagrams point to environmental impacts being highest from using elements that are both in high demand and which have low sustainability indices. Modelling that emphasises the supply chain, environmental assessment and social indicators can provide measures of various types of risks in the industrial usage of elements, as in the case study summarised in Table 2.22
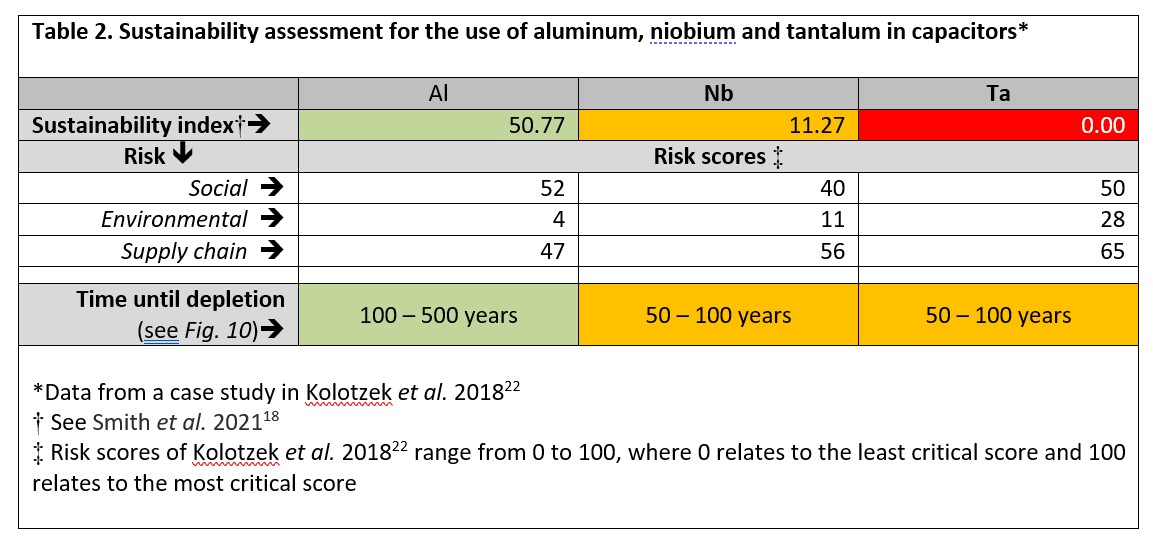
In the 1800s, the English Romantic poet, William Wordsworth unknowingly may have foreshadowed the Taoist yang of the current situation on Earth perfectly:
“The world is too much with us; late and soon,| Getting and spending, we lay waste our powers;—| Little we see in Nature that is ours; | We have given our hearts away, a sordid boon! |This Sea that bares her bosom to the moon;| The winds that will be howling at all hours,| And are up-gathered now like sleeping flowers;| For this, for everything, we are out of tune; |It moves us not…”23
Although all of the periodic tables shown in this chapter reveal chemical elements that have yin or yang characteristics, some elements such as carbon are both yin and yang. Carbon in the form of organic molecules was important to our and all of Earth’s lifeforms’ evolution, and remains important for our continued existence – and thereby it is yin. And yet carbon is also at the heart of our historic and current demand for energy, whether in its own right for fuel for warmth, light and transport or indirectly for industrial processing to satisfy other of our needs and wants – and thereby it is yang as well.
The yin and yang of carbon and the hydrogen economy
Exploitation of carbon, whether in the form of coal, natural gas or petroleum, releases carbon dioxide if the material is burned - a significant 'greenhouse’ gas. Reduction in the exploitation of carbon for energy purposes in the short term seems unlikely, so real reduction of emissions of gaseous carbon dioxide are unlikely to be achieved. Removal of carbon dioxide by 'sequestration’, i.e., removing it from exhaust gases or from the atmosphere and incorporating into a solid compound has been suggested as an alternative. An approach being used in Iceland is to pump the carbon dioxide “into underground caverns where the gas, mixed with water, will slowly become stone as it cools”.24 Experiments have found that the pumped-in gas turned to carbonate within two years rather than taking the hundreds or thousands of years thought to be necessary.25 In fact, the ‘underground caverns’ are in basaltic rocks, which other research has shown can react with carbon dioxide:
“…the formation of solid carbonate minerals (calcite, magnesite, dolomite, and a variety of hydrated Mg-carbonate minerals such as nesquehonite) through reaction of CO2 (gas, liquid, dissolved in water, or supercritical) with rocks rich in calcium or magnesium. The best sources of Mg and Ca are mafic and ultramafic rocks (mantle peridotite, basaltic lava, and ultramafic plutons), mine tailings (mafic and ultramafic), and industrial byproducts (cement kiln dust, steel slag, and fly ash).”26
Whether such technology could counter the current or likely future excess of carbon dioxide in the atmosphere can be debated, but philosophically, the change of carbon (in the form carbon dioxide) as villain – i.e., yang –in climate change to carbon (in the form of a carbonate) as an environmental saviour – i.e., it becomes yin – can be considered as a demonstration of the endless cyclic movement that characterises the Tao:
To shrink something | You need to expand it first | To weaken something | You need to strengthen it first | To abolish something| You need to flourish it first | To take something | You need to give it first.27
Consistent with this, social psychologist Richard Nisbett asserted in 2003 (p. 174)28 that, in Eastern thought, nature is constantly changing: “being in a given state is just a sign that the state is about to change. Because reality is in constant flux, the concepts that reflect reality are fluid and subjective rather than being fixed and objective,” and that “complexity and interaction meant for the [ancient] Chinese that an attempt to understand the object without appreciation of its context was doomed” (p. 19). In essence, the inter-dependence of all things in the environment – including people – was accepted by Easterners, but a belief in the controllability of the environment was more characteristic of Westerners than Easterners.
A pervasive theme in Nisbett (2003) is that both Eastern and Western ancient thought has persisted to the present, which concurs with the identification by Joseph Needham (a Eurocentric doyen of Chinese philosophy) of:
“a complete gulf between theoretical scientific thought and technical human practice for thousands of years. Aristotelian and Epicurean speculations such as the atomic theory went their way without any influence on actual techniques, while the Chinese scholars, masters of the ideographic characters, but remote from their own technical artisans, continued until a late date to harp on the primitive theories of the five elements and the two principles; the yang and the yin.”29
Clearly this is no longer the case: the resurgence of China as an economic powerhouse has meant that China, the so-called ‘tiger’ economies of eastern Asia and the other developing countries are as energy-hungry and polluting as the Western nations that they are quick to decry. Despite the development of alternatives to carbon (from coal, oil, and gas), “every datapoint showing the speed of change in energy can be countered by another showing the stubbornness of the status quo”.30 That said, recent research suggests that relatively cheap metals, e.g. aluminium, can be used as catalysts in Fischer Tropsch chemistry - an industrial process that transforms carbon monoxide into liquid hydrocarbons (Eq. 1).31 Although development of the Fischer Tropsch process does not address global warming because hydrocarbons are still produced, it improves outcomes of column 6 (Energy) and column 8 (Catalysis) of Fig. 6.
(2n + 1)H2 + nCO → CnH(2n+2) + nH2O (Eq. 1)
where n is an integer
For n=1, the product is methane, an undesirable by-product in most gas-to-liquid and coal-to-liquid applications. Accordingly, the reaction conditions are designed to maximise the formation of hydrocarbon liquid fuels of higher molecular weight.
In the longer term the so-called ‘hydrogen economy’ appears to offer an alternative to hydrocarbon-based energy sources. This is hardly a new idea: physical chemist John Bockris suggested it as far back as the early 1970s.32 Despite optimism in the early 2000s,33 there was subsequent criticism of the idea,34 and even now support is somewhat guarded:
“… the major driver affecting hydrogen cost is the characteristics of the source from which hydrogen is extracted. In 2008 approximately 96% of Hydrogen produced in the world came from fossil fuels and the share hasn't changed significantly since then. Ironically this doesn't do much to solve the global warming problem and exacerbates the carbon cycle…. However, hydrogen obtained via renewable energy powered electrolysis allows for better well-to-wheel efficiencies and potentially overall reduced fuel cost. Hindering progress in realising this at present is the development challenge of carrying out electrolysis of water at practical efficiencies and scale. It must be said though that if the cost of renewable energy power generation continues to fall, then it is expected the cost of hydrogen produced from electrolysis to reduce as well.”35
The essence of the hydrogen economy requires revisiting the water-gas shift reaction, sometimes abbreviated to WGSR (Eq. 2). Industrially important, this reaction traditionally uses high-temperature catalysts to 'push’ the reaction between carbon monoxide and water towards the products carbon dioxide and hydrogen:
CO +H2O → CO2 + H2 (Eq. 2)
In a variant of this reaction, hydrogen can be produced from natural gas, as shown below for methane:
CH4 + H2O → CO +3H2 (Eq.3)
CO + H2O → CO2 + H2 (Eq.4)
CH4 + 2H2O → CO2+ 4H2 (Eq.5)
The hydrogen produced in this way is sometimes referred to as ‘blue hydrogen’.
Other ‘feedstocks’ such as petroleum (in which case the hydrogen produced is sometimes referred to as ‘grey hydrogen’) or biomass can be used. Recent research suggests that catalysts working at low temperatures using gold on molybdenum carbide, in particular,36 joins other techniques being developed that make this reaction more effective and efficient.37 Perhaps unsurprisingly, given the current concern about the increasing levels of carbon dioxide in the Earth’s atmosphere, there is also interest in using the reverse water gas shift reaction, sometimes abbreviated to ‘RWGS’ (Eq. 6) to recycle carbon.38
CO2+ H2 → CO + H2O (Eq. 6)
This would require abundant hydrogen to be available, perhaps by the electrolysis of water (Eq. 7) using electricity generated from solar power and being a combination of the two half-reactions given by Eqs. 8 and 9. The hydrogen produced in this way is sometimes referred to as ‘green hydrogen’.
H2O → H2 + ½ O2 (Eq. 7)
2H2O → O2 + 4H+ + 4e- (Eq. 8)
2H+ + 2e- → H2 (Eq. 9)
Alternatively, or at least as an interim strategy, the sequestration techniques previously described could be used in association with the conventional water gas shift reaction that would produce a fuel – hydrogen –and to assist in removing carbon dioxide from the atmosphere, according to Eq. 5 followed by Eq. 10.
2CO2+ M2SiO4 → 2MCO3 + SiO2 (Eq. 10)
where M = Mg, M2SiO4 represents olivine and MCO3 represents magnesite.
where M = Ca, M2SiO4 represents calcium orthosilicate and MCO3represents calcite.
Conclusions
The examples of carbon and hydrogen discussed here suggest that for planet Earth a Taoist compromise would provide a balance between the yang of the combined environmental concerns of Fig. 10 with the yin of green chemistry and engineering referred to earlier, extending beyond the energy initiatives.39 Moreover, despite our increasing current dependence on the scarce metals and metalloids of the middle part of the periodic table for continued technological advancements, it appears that it will be the abundant elements of the upper part of the periodic table that will have the most critical role to play in our- if not our planet’s – survival, as suggested by the fragment of the periodic table shown in Fig. 11.
As discussed, carbon has a central part to play in this ‘greening’: changing from being perceived as a yin element in the 19thcentury, when the beauty of biology was centre-stage,40 to a yang element when it was attributed a prime role in the evolution of ‘us’ and its incorporation into organic molecules for medicines, plastics, etc. seemed unlimited,41 and potentially becoming yin again if carbon can be constrained by human effort to minimise the effect of over a century’s anthropogenic emissions of carbon dioxide.42 However, science may not be enough on its own to achieve this. Although most scientists and non-scientists apparently accept the validity of global warming,43 that alone does not change attitudes:44 for the public at least, personalising both the problem and the solution may help in achieving this change.45

Thinking yet more widely, an assessment of the value of Needham’s decades of research into Chinese philosophy extends the notion of compromise, advocating “a synthesis of Eastern tradition and Western thought”, that might yet address his concern that with “the spread of neo-liberalism and the alienation of democratic systems, there has been a resulting increase in political disempowerment, economic inequality, and environmental degradation” (Grinter 2018).46 Grinter is certainly not alone in drawing attention to wider social and economic matters.47 Perhaps further thinking that complements green chemistry and green engineering might be stimulated by this paper.
References and notes
1. Lao Tzu. Tao Te Ching, trans. Ch’uTa-Kao. Allen & Unwin: London, 1970, chapters 25 and 40.
2. Reference 8 in Chinese thought: Wang Ch’ung ad 80, quoted in Needham, J. Science and Civilisation in China. Cambridge University Press: London, 1956, vol. IV, p. 7.
3. Capra, F. The Tao of Physics. An Exploration of the Parallels Between Modern Physics and Eastern Mysticism. Bungay (United Kingdom): Fontana / Collins, 1977, p. 112-113.
4. Graham, A.C.: Disputers of the Tao: Philosophical Argument in Ancient China. Open Court: La Salle (Illinois), 1989, p. 331.
5. Graham 1989, op. cit., pp. 335-336.
6. Kuel Ku Tzu, 4th century bc, quoted in Needham, J. Science and Civilisation in China. Cambridge University Press: London, 1956, vol. IV, p .6; also cited in Capra 1977, op. cit., p. 114.
7. Kaleağasioğlu, F. Conte’s approach to human cognition by application of quantum mechanics: a revisitation of the Yin-Yang theory and Jung’s psyche model by using such quantum approach. Neuroquantology 2012,10 (4), 655-662.
8. Toxic and Essential Elements. Fromhttps://www.doctorsdata.com/resources/pdfs/DDI_Toxic_and_Essential_Elements_Brochure.pdf (accessed 10/01/2022).
9. Railsback, L.B. The earth scientist’s periodic table of the elements and their ions: a new periodic table founded on non-traditional concepts. In Scerri, E.; Restrepo, G. (Eds). Mendeleev to Oganesson: A multidisciplinary perspective on the periodic table. Oxford University Press: New York, 2018, pp. 206-218.
10. Kyle, J.H.; Breuer, P.L.; Bunny, K.G.; Pleysier, R.; May, P.M. Review of trace toxic elements (Pb, Cd, Hg, As, Sb, Bi, Se, Te) and their deportment in gold processing. Part 1: Mineralogy, aqueous chemistry, and toxicity. Hydrometallurgy 2011, 107 (3-4), 91-100.
11. This is also implied in Scerri, E. The Tao of chemistry. J. Chem. Ed.1986, 63 (2), 106-107.
12. Anastas, P.T.; Zimmerman, J.B. The periodic table of the elements of green and sustainable chemistry. Green Chem. 2019, 21, 6545-6566. The material has also been published as a free book, available online: Anastas, P.T.; Zimmerman, J.B. The periodic table of the elements of green and sustainable chemistry. Press Zero: Madison, Connecticut, 2020. https://greenchemistry.yale.edu/sites/default/files/files/Book%20-%20Periodic%20Table%20of%20the%20Elements%20of%20Green%20and%20Sustainable%20Chemistry.pdf (accessed 05/04/2023).
13. Astruc, D. The metathesis reactions: from a historical perspective to recent developments. New J. Chem. 2005, 29, 42-56.
14. Casey, C.P. [The] 2005 Nobel Prize in chemistry. Development of the olefin metathesis method in organic synthesis. J. Chem. Ed. 2006, 83 (2), 192-195, https://doi.org/10.1021/ed083p192 ; see also: The Nobel Prize in Chemistry 2005. https://www.nobelprize.org/prizes/chemistry/2005/summary/ (accessed 04/05/2023).
15. Cespi, D.; Esposito, I.; Cucciniellio, R.; Anastas, P.T. Beyond the beaker: benign by design society. Curr. Res. Green Sustain. Chem. 2020, 3, June 2020, article 100028.
16. Marcelino, L.; Sjöström, J.; Marques, C.A. Socio-problematization of green chemistry: Enriching systems thinking and social sustainability by education. Sustainability 2019, 11 (24), article 7123 https://doi.org/10.3390/su11247123.
17. De Marco, B.A.; Rechelo, B.S.; Tótoli, B.G.; Kogawa, A.C.; Salgado, H.R.N. Evolution of green chemistry and its multidimensional impacts: A review. Saudi Pharm. J. 2019, 27 (1), 1-8.
18. Smith, L.; Ibn-Mohammed, T.; Reaney, I.M.; Koh, S.C.L. A chemical sustainability index. Resources, Conservation and Recycling 2021, 166, https://doi.org/10.1016/j.resconrec.2020.105317.
19. Zafsar, S. The environmental benefits of using titanium. Bioenergy Consult, 2021. https://www.bioenergyconsult.com/titanium-an-environmental-vanguard/ (accessed 04/05/2023).
20. Supanchaiyamat, A.; Hunt, A.J. 2018.Conservation of critical elements of the periodic table. Chem. Sustain. Energy Mat. 2018, 12 (2), 397-403, https://doi.org/10.1002/cssc.201802556.
21. Nuss, P.; Eckelman, M. Life cycl eassessment of metals: A scientific synthesis. PLOS One 2014, 9(7), e191298, http://dx.doi.org/10.1371/journal.pone.0101298.
22. Kolotzek, C., Helbig, C., Thorenz, A., Reller, A., Tuma, A. A company-oriented model for the assessment of raw material supply risks, environmental impact and social implications. J. Cleaner Prod. 2018, 176, 566–580. Cited in: Smith, L.; Ibn-Mohammed, T.; Koh, L.; Reaney, I.M. Life cycle assessment of functional materials and devices: Opportunities, challenges, and current and future trends. J. Am. Ceram. Soc. 2019, 102 (12), 7037-7064, https://doi.org/10.1111/jace.16712.
23. Wordsworth W. The world is too much with us. Poems, in Two Volumes. Longman, Hurst, Rees, and Orms: London, 1807. The poem was written in ca. 1802.
24. World’s largest carbon capture plant opens in Iceland. 2021, September 9. https://www.smithsonianmag.com/smart-news/worlds-largest-carbon-capture-plant-opens-iceland-180978620/ (accessed 13/05/2022).
25. Iceland carbon capture project quickly converts carbon dioxide into stone. 2016, June 9. https://www.smithsonianmag.com/science-nature/iceland-carbon-capture-project-quickly-converts-carbon-dioxide-stone-180959365/ (accessed 12/05/2022).
26. Keleman, P.; Benson, S.M.; Pilorgé, H.; Psarris, P.; Wilcox, J. An overview of the status and challenges of CO2 storage in minerals and geological formations. Front. Climate 2019, 15 November, article https://doi.org/10.3389/fclim.2019.00009.
27. Tao Te Ching, Chapter 31, cited in: Nisbett, R.E. The Geography of Thought: How Asians and Westerners Think Differently … and Why. The Free Press: New York, 2003, p. 14.
28. Nisbett, R.E. The Geography of Thought: How Asians and Westerners Think Differently … and Why. The FreePress: New York, 2003, p. 19 and p. 174.
29. Needham, J. The Grand Titration: Science and Society in East and West. Taylor and Francis, 2005, p. 141-142.
30. World Energy Outlook, 2021. https://www.iea.org/reports/world-energy-outlook-2021 (accessed 12/05/2022).
31. Coles, M.P. A decade of main group chemistry research. Chem.NZ 2022, 86 (3), 115-125; Coles, M.P. The periodic table is my molecular Lego. Inaugural lecture, Victoria University of Wellington, 14 June 2022, https://www.wgtn.ac.nz/events/2021/10/inaugural-lecture-the-periodic-table-is-my-molecular-lego (accessed11/07/2022).
32. Bockris, J. O’M. A hydrogen economy. Science 1972, 176 (4041), 1323. Four decades later Bockris provided ahistoric context for his ideas in: Bockris, J. O’M. The hydrogen economy: Its history. Int. J. Hydrogen Energy 2013, 38 (6), 2579-2588.
33. Barreto, L.; Makihira, A.; Riahi, K. The hydrogen economy in the 21st century: a sustainable development scenario. Int. J. Hydrogen Energy 2003, 28 (3), pp. 267-284.
34. Flood, W. The hydrogen economy – global solution or pie in the sky? Energy Env. 2012, 23 (6-7), 1097-1104.
35. Tashie-Lewis, B.C.; Nnabuife, S.G. Hydrogen production, distribution, storage and power conversion in a hydrogen economy – A technology review. Chem. Eng. J. Adv. 2021, 8, article 100172, https://doi.org/10.1016/j.ceja.2021.100172.
36. Yao, S.; Zhang, X.; Zhou, W. et al.Atomic-layered Au clusters on α-MoC as catalysts for the low-temperature water-gas shift reaction. Science 2017, 357 (6349),389-393, https://doi.org/10.1126/science.aah4321.
37. Diaz, E.; Ordóñez, S. The role of heterogeneous catalytic processes in the green hydrogen economy [Editorial]. Catalysts 2021, 11, 1185. https://doi.org/10.3390/catal11101185.
38. Brown, R.M.; Joyce, M.; Zhang, Q.; Reina, T.R.; Duyar, M.S. Identifying commercial opportunities for the reverse water gas shift reaction. Energy Technol. 2021, 9 (11), https://doi.org/10.1002/ente.202100554.
39. Gielen, D.; Bodshell, F.; Saygin, D.; Bazilian, M.D.; Wagner, N.; Gorini, R. The role of renewable energy in the global energy transformation. Energy Strategy Rev. 2019, 24,38-50.
40. Hibberd, S. The atom of charcoal. In: Brambles and Bay Leaves: Essays on the Homely and Beautiful. London, 1865.Cited in Maybey, R. The Cabaret of Plants. Botany and the Imagination. Profile: London, 2015, p. 183.
41. Hodder, A.P.W.; Hume, A.; Jenks, A.; Peters, J. Keeping track of Charlie. Earthworks Book A – Planets Beyond Earth. User Friendly Resources: Christchurch (New Zealand), 1999, p. 18.
42. Zimmer, Verdict (almost) in. Discover Magazine, 1996. [Reprinted in: McKinney, M.L.; Tolliver, R.L.; Shariff, P. (eds), Current Perspectives in Geology. Wadsworth: Belmont (California), 1998, p. 178-179.
43. Ramanujan, K. More than 99.9% of studies agree: Humans caused climate change. Cornell Chronicle 2021, October 19, https://news.cornell.edu/stories/2021/10/more-999-studies-agree-humans-caused-climate-change (accessed 16/07/2022).
44. Weber, E.U. What shapes perceptions of climate change? New research since 2010. WIREs Climate Change 2016,7, 125–134, https://wires.onlinelibrary.wiley.com/doi/abs/10.1002/wcc.377
45. D’Alfonso, T. The element of surprise: New Australian documentary rebrands carbon 2022. https://popcornpodcast.com/features/carbon-the-unauthorised-biography (accessed 04/05/2023); see also: Carbon: The Unauthorized Biography. 2022. https://www.cbc.ca/natureofthings/episodes/carbon-the-unauthorized-biography (accessed 04/05/2023).
46. Grinter, A. The grand titration: Revisiting the work of Joseph Needham to address ethnocentrism in contemporary philosophy and society. Cosmos History: J. Natural Social History 2018, 14 (3), https://www.academia.edu/37990214/The_Grand_Titration_Revisiting_the_Work_of_Joseph_Needham_to_Address_Ethnocentrism_in_Contemporary_Philosophy_and_Society (accessed 04/05/2023).
47. Examples include: Moyo, D. Edge of Chaos. Why Democracy is Failing to Deliver Economic Growth – and How to Fix it. Little Brown: London, 2018; Wolf, M. The Crisis of Democratic Capitalism. Allen Lane: London, 2023. [Wolf’s book is reviewed in: Runciman, D. But how? London Review of Books 2023, 45(7, March 30), 23-24.]